|
Wavelength Dispersive Spectrometers |
|
Wavelength dispersive spectrometers differentiate X-rays based on their wavelength. They are mechanical systems consisting of two main components, an analyzing crystal and a detector. They work on the principle of X-ray diffraction. When the electron beam hits the sample, X-rays are produced. A portion of those X-rays will hit the analyzing crystal in each of the spectrometers. Those X-rays which have the right wavelength will diffract into the detector, where they will be counted. For the diffracted X-rays to enter the detector the spectrometer must be properly aligned. This means that the detector, analyzing crystal, and the spot on the sample where the X-rays are generated, must all be on the circumference of a circle, called the Rowland circle. Under these conditions only the X-rays with the wavelength of interest will be counted by the detector. To measure X-rays with a different wavelength, the analyzing crystal and detector must be rotated around the Rowland circle such that the incident angle of the crystal changes, while maintaining position on the Rowland circle. In practice, the crystal is moved directly away from the sample and rotated, and the detector is moved around on the circumference the circle. As a result, the center position of the Rowland circle changes, but its diameter stays the same. The position of the spectrometer can be given in terms of:
1) the wavelength of the X-rays it is collecting,
2) the diffraction or incident angle, or
3) the distance between the sample and the crystal
(L-value).
|
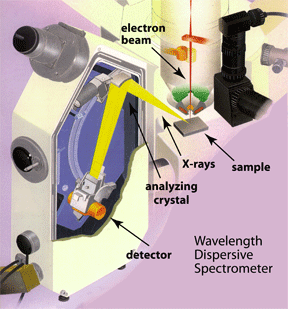
JEOL Wavelength Dispersive Spectrometer
|
|
|
|
|
Regularly spaced atoms in a crystal structure can diffract X-rays. This means that X-rays hitting a crystalline material will re-radiate in a particular direction under certain conditions. The direction and conditions are defined by Bragg's law. The parameters that define whether diffraction will occur are the wavelength of the X-rays, the incident angle of the X-rays, and the interplanar spacing within the crystal. If diffraction occurs, the X-rays leaving the crystal will be in phase, and the departure angle will be equal to the incident angle.
A geometric explanation for this phenomenon can be seen in the diagram to the right. The lower ray in each figure must travel a farther distance than the upper. If the extra distance (A-B-C) is exactly an integer number of wavelengths (1,2,3 ...) then the two X-rays will leave in-phase. If the distance A-B-C is not an integer number of wavelengths, the two rays will be out of phase, destructive interference will occur, and the X-rays will be absorbed by the material. One can see that for a given d-spacing (the interplanar spacing of the crystal), the distance A-B-C can be changed by changing the incident angle.
In a wavelength dispersive spectrometer, the incident angle can be changed by moving the crystal around the Rowland circle. Therefore at each position a different wavelength of X-rays will diffract, and only those X-rays that diffract will enter the detector. Because of the physical limitations of the spectrometers, no single crystal can diffract all of the X-rays produced from the elements in the periodic table. Each crystal will only be able to diffract a certain range of wavelengths. Therefore WD spectrometers will have a number of crystals to choose from depending on the elements being analyzed. |
|
|
|
|
There are two types of detectors commonly in use, the Xenon counter (XPC) and the gas flow proportional counter (GFPC). Both operate from the same basic principle.
The counter itself is a cylinder filled with a gas. The gas is separated from the vacuum of the instrument by a thin window. The X-ray photon enters through this window where it knocks out electrons from the electron shells of the gas molecules. These electrons are attracted to a wire running down the center of the detector, which has a positive 1-3 kV potential on it. The electrons hitting the wire produce a current which is picked up by the preamp and then turned into a signal. The amount of current is proportional to the number of X-rays that are entering the detector.
|
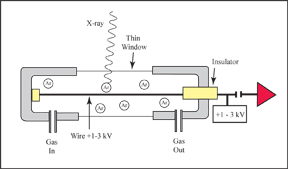
Gas flow proportional counter
(after Lee, R.E., 1992).
|
|
|
In the Xenon counter, the gas in the cylinder is Xenon, and the cylinder is sealed. The window that separates the detector from the vacuum is beryllium. Because the window is made of beryllium, low-energy X-rays from light elements can get absorbed. Therefore this type of detector is only used in spectrometers with LIF and PET crystals, where higher energy X-rays will be analyzed.
For low-energy X-ray work, the gas flow proportional counter will give a higher count rate. The windows on these counters are made from a thin polymer. They are thin enough to allow the low-energy X-rays to enter; yet they are strong enough to withstand the difference in pressure between the vacuum and the atmospheric pressure inside the counter. However, they are thin enough that the gas will slowly leak through the membrane. To over come this, these counters have the gas flowing through them continuously. The gas used is P10, a mixture of argon and methane. |
|
|
|
|
|
No single type of crystal is able to diffract over the entire range of wavelengths that are needed to cover all of the elements in the periodic table. Each type of crystal has a fixed d-spacing, and therefore has a fixed range of elements that it will be able to detect. Table 1lists commonly used crystals.
The TAP, PET, and LIF crystals cover most of the periodic table with the exception of the light elements (Table 2). Their names are abbreviations of their compositions.
• TAP = thallium acid phthalate
• PET = pentanerythritol
• LIF = lithium fluoride
LDE crystals are used for light elements. They are not actually crystalline materials, but are layered synthetic microstructures. They are manufactured by depositing alternating layers of heavy and light elements (like carbon and tungsten). The layers are very thin, on the order of 50-150 A, and act like lattice planes in a crystal structure. Since they are manufactured, the LDE crystals can be fine tuned to diffract specific elements. The listed d-spacings in Table 1 for the LDE crystals are approximates only, as each crystal is slightly different. |
Table 1. Comparison of the commonly
used crystals.
Crystal |
2d (nm) |
Comment |
TAP |
2.576 |
- |
TAPH |
2.576 |
for H-spect |
PET |
0.8742 |
- |
PETH |
0.8742 |
for H-spect |
LIF |
0.4027 |
- |
LIFH |
0.4027 |
for H-spect |
LDE1 |
~ 6 |
N, O, F |
LDE2 |
~ 10 |
C |
LDEB |
~14.5 |
Be, B |
LDE1H |
~ 6 |
for H-spect |
LDE2H |
~ 10 |
for H-spect |
LDENH |
~ 8 |
for H-spect |
LDE3H |
~ 20 |
for H-spect |
LDE5H |
~ 8 |
for H-spect |
LDEBH |
~14.5 |
for H-spect |
|
|
|
Table 2. Range of elements that can be analyzed by the TAP, PET, and LIF crystals. |
|
|
|
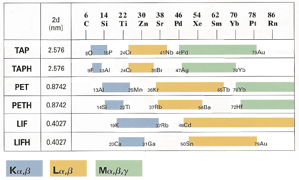 |
|
|
References: Lee, R.E., 1992, Scanning Electron Microscopy and X-ray Microanalysis. Prentice Hall, 458p. |
|
|
|
|